Production of recombinant mammalian proteins
Proteins and metabolic products from animal cells are an important target for biotechnologists, with important applications in medicine and, to a lesser extent, food. We are now more aware of the dangers of using mammals as direct sources of protein and fortunately the days of using human cadavers as sources of therapeutic hormones are over. An example of this is insulin, which before 1982 was extracted from cows or pigs for use in diabetes therapy. However, yields were low and a minority of patients developed an immune response to the injections. These patients could have been given insulin from human sources, but the risk of immune reaction from co-purified proteins remained, along with the possibility of contracting diseases such as hepatitis (or latterly AIDS). The development of Humulin (human insulin expressed in E. coli, developed from 1978 by Genentech) surmounted some of these problems, and this is generally held to be the first recombinant Bacterial bioproduct used in medicine.
For a human gene to be expressed in a bacterium such as E. coli, a number of precautions must be taken to ensure that the resulting protein is similar to the human equivalent. The gene must be free of introns, as Bacteria use different signals for post-transcriptional processing. The most efficient way of doing this is to use human cDNA, made by the action of reverse transcriptase on a messenger RNA sample. For more details of cDNA synthesis, see Instant Notes in Molecular Biology. Once the introns are removed from the gene, it may also be necessary to use site-directed mutagenesis to change which codons are used by the bacterium during translation – the set of codons used by humans is not identical to that used by E. coli, and absence of a codon-specific tRNA can lead to lower yields, random insertion of amino acids or a complete cessation of translation.
Once the gene has been adapted to function in a Bacterial cell, it can be placed under the control of a suitable promoter and production of the recombinant protein can start. A list of some of the proteins that have been engineered in this way appears in Table 1. However, even though the recombinant protein has the identical amino acid sequence to its human counterpart, the product may not have the same activity during therapy as the native drug target. There may be a number of reasons for this, as follows:
? It is not possible to make an absolutely pure preparation of any protein – a contaminating bacterial protein may be causing inhibition or immune reaction.
? Bacterial bioproducts may be contaminated with bacterial lipopolysaccharides (Section C8), which are toxic.
? The protein may only function properly when a certain number of sugar molecules are attached (when the protein is glycosylated). Bacteria use different signals for glycosylation than human cells.
Table 1. A selection of human proteins cloned in E. coli and their therapeutic use
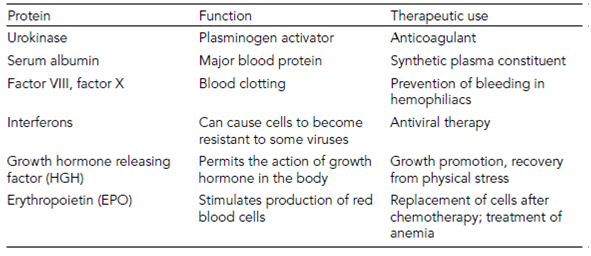
Glycosylation problems mean that Bacteria are not always suitable for recombinant expression. Yeast or fungi can be used, and although the number of sugar molecules increases over Bacteria, glycosylation still will not be identical. An example of this is recombinant erythropoietin (EPO), which is used to boost red blood cell counts in leukemia and other cancer patients. Bacterial EPO needs to be administered in much higher doses than that produced from human cadavers, and the difference in glycosylation is used as a test for EPO’s illegal nontherapeutic use as a blood booster in endurance sports.
Few mammalian recombinant proteins are used outside of medicine, but a few commonly used enzymes are produced in this way. Recombinant bovine rennin provides a way of making curd for cheese that is suitable for vegetarians and is also consistent in quality, avoiding its collection from calves’ stomachs.