Visual attention
Attention selectively filters out the irrelevant information in favor of the relevant that may be consciously perceived. When this occurs to sensory information it is known as sensory attention and this has been extensively deliberate in vision.The visual stimuli can be attended to by moving the head or eyes, but attention to any stimuli can also be made without moving, by modifications to the internal state. Covert visual attention shifts separately of eye movements. It can be studied in humans and primates by spatial cueing tasks.
The attentional methods are thought to include increasing the signal-to-noise ratio and increased gain of those neurons which encode the selected representation. The neural correlates of this include increased firing frequency, greater synchronicity in firing or synaptic activity in neuron populations, decreased response variability, and transient modulation of receptive fields. These sorts of variable can bestudied in conscious humans and by electrophysiology in awake, behaving animals.
For illustration, the visual attention is accompanied by modifications in the receptive field properties of few visual neurons. During the attentional tasks these cells become transiently biased to ignore distractor stimuli which would, in the non-attending state, elicit a response. It is as when in attention the RFs shrink to encompass only the target stimulus, therefore the cell responds to it alone. This is rising of the signal-to-noise ratio. Not all the visual system neurons are modulated in visual attention. Only the cells with large sufficient RFs to respond to numerous objects are included. These are normally found in areas responsible for late instead of early visual processing.
Though, receptive field properties position constraints on visual attention. For illustration, the target objects which differ in apparent depth and movement from the surrounding distractors “pop out” as there are visual system neurons which encode both the binocular disparity and movement. In contrast, the cells which respond to both the movement and color have never been specified and the visual system is presumably forced to analyze each object in turn for each of these stimulus features in order to specify the one with the target combination; this takes time.The circuitry implicated in visual attention is closely associated to that included in oculomotor control as shown in figure below. In monkeys, the stimulation of FEF at levels is too low to generate saccades enhanced performance in an attention task. The same effects have been found for the superior colliculus and regions of parietal cortex. The other studies illustrate that activation of the FEF not only permits covert attention to the proper location in the visual field but also selects the suitable saccades to fixate the target; therefore visual attention and oculomotor control are tightly linked. Numerous cortical regions generate attentional signals (V4, V2, V1, lateral prefrontal cortex) however the earliest activity in attentional tasks is seen in the FEF that implies that this is the source of attention-control signals.
Significant evidence associates three thalamic nuclei, the pulvinar, LGN, and thalamic reticular nucleus in the visual attention. The electrophysiology on behaving monkeys, brain imaging, and lesions all support a role for pulvinar in controlling the visual attention at a cortical level. It gets input from the superior colliculus, LGN, & cortex, has topographic maps of the visual world and responds selectively to different aspects of visual stimuli involving color, orientation, and motion, presumably as of its cortical connections. Motion-sensitive pulvinar neurons react to movement in the real world but are silent for the apparent motion of a stimulus caused by eye movements as input from the superior colliculus permit them to subtract retinal signals formed by eye movements. The pulvinar connections with the cortex are widespread, involve visual cortex, and are reciprocal. As a common rule if two regions of cortex are directly connected they are also indirectly connected through the pulvinar. In this manner the pulvinar is thought to modulate the efficacy of direct inputs from one cortical region to another. This can be an attention mechanism.
Though, the pulvinar might do more than this. It also controls the intrinsic oscillations of cortical neurons and therefore could synchronize firing of broadly distributed cortical neurons representing various aspects (color, form, depth, motion) of similar object. This could be how the brain integrates information in parallel visual channels to generate a unified percept: a solution to the binding problem. The pulvinar lesions in humans can disturb binding of the visual features.
Human brain imaging displays that visual attention enhances LGN responses to attended positions and attenuates those to ignore the stimuli. There seem to be two routes by which this occurs, with various functions:
- The thalamic reticular nucleus (TRN) has access to information from the visual cortex, prefrontal cortex, and superior colliculus, and makes retinotopic connections with the LGN. The TRN is inhibitory on the LGN and attention correlates with reduce in this inhibition. This route seems to be responsible for an early LGN response that is thought to be attention to a particular location in the visual field.
- The back projections from the FEF through V4 and V1 are retinotopic and enhance LGN responses. In this route, this is responsible for the next phase of attention (200 ms after a stimulus looks), P, M, & K streams are segregated therefore could allow particular features (example, form, motion) to be selected for attention even at the early LGN processing stage.
Therefore the LGN acts as a “gatekeeper” selecting what retinal input is transmitted via to the visual cortex.
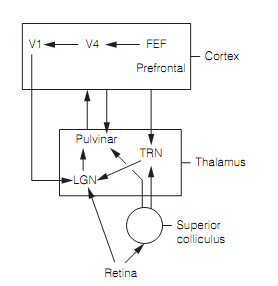
Figure: Circuitry implicated in visual attention. The pulvinar makes reciprocal connections with many cortical regions. Here, FEF=frontal eye fields; LGN=lateral geniculate nucleus; and TRN=thalamic reticular nucleus.